A flexible radio frame structure
In 4G and 5G, transmissions and receptions in the radio spectrum are coordinated by time unit. This unit of time, called a radio frame, has a duration of 10ms in both technologies, and is further subdivided into 10 subframes of 1ms each, as shown in the figure below:

In 4G, as we saw in the episode about spectral efficiency, these subframes are the fundamental temporal unit for allocating resources in the upstream and downstream directions.
In 5G, things are less fixed. In fact, the frequency spacing between sub-carriers (SCS) is variable, its value being given by 2µ.15 kHz , where µ is called "numerology". This flexibility allows better adaptation to wider frequency bands and implies higher data rates on the sub-channels and therefore the ability to transmit more information per unit of time, as illustrated in the following figure:
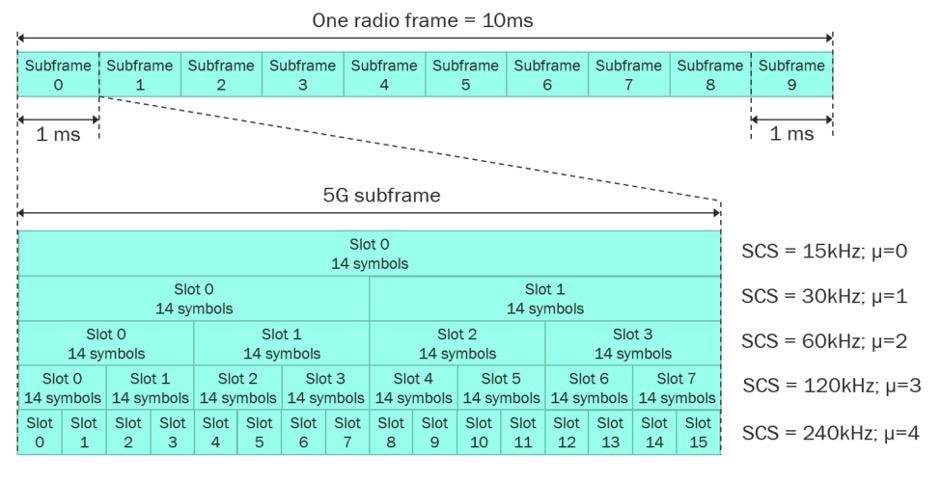
In this case, the fundamental time unit to be considered for resource allocation is the slot, and its duration varies from 1ms to 62µs depending on the numerology used.
So, since the time it takes for information to travel across the radio interface is directly dependent on the ability to allocate a radio resource to transmit it and on the transmission time itself, we can say that for systems loaded in an equivalent way, the latency in one direction in 5G is divided by a multiple of 2µ where µ is the numerology used.
The mini-slot concept
To further improve latency, 5G has introduced the concept of the mini-slot. As shown in the figure below, a standard 5G slot comprises 14 OFDM symbols. A mini-slot is a resource time unit comprising a variable number of OFDM symbols (2, 4, 7) and can start at any position within a standard slot.

This mechanism, controlled by parameters K, S and L as illustrated above, increases transmission opportunities (they are shorter and several transmissions can be scheduled in the same slot) and allows them to start instantaneously without waiting for the next slot boundary. It is particularly well suited to applications where there is little information to transmit, such as thecontrol of industrial PLCs.
Flexible slot formats
The latency perceived by the end user (latency at application level) also depends on the efficiency of interactions between transmitters and receivers. Thus, the way in which radio frames are organized between the upstream direction (terminal to network) and the downstream direction (network to terminal) is a very important factor to take into account.
In 4G, the organization of TDD frames is governed by 7 different formats as shown in the following figure:
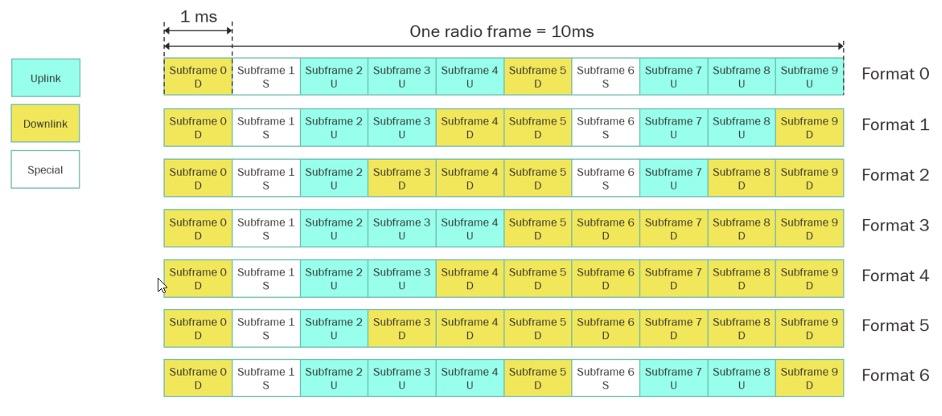
D frames can be used by the network to transmit information to a terminal, while U frames can be used by a terminal to reply to the network. S frames are special frames used to reserve a guard time between terminal reception and transmission. This guard time depends on the size of the cells and the terminal's reaction time capacity.
Formats 0, 1 and 2 are reserved for private networks in France. Format 2 is imposed on public networks by the French regulatory authority for electronic communications, post and press distribution (ARCEP).
In 5G, things are once again very flexible: the organization of the TDD frame is not fixed by a rigid format as in 4G, but by a set of configuration parameters communicated by the network to the terminal:
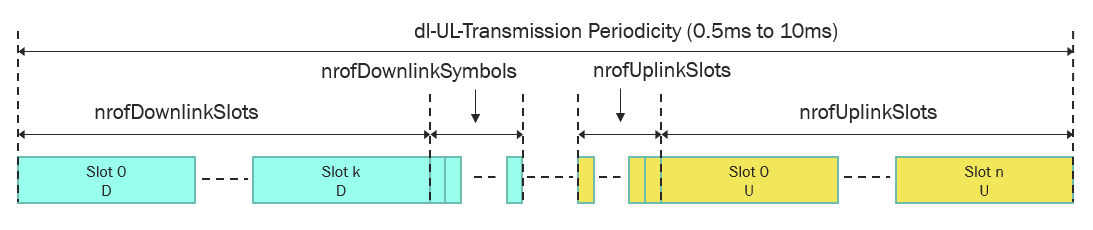
Thus, in 5G, it is much easier to adapt the radio frame to use cases, in order to harmoniously distribute reception and transmission opportunities with a short periodicity to guarantee fluid interactions between terminals and the network. It shall be noted that public 5G networks cannot exploit this flexibility, as they are subject to the same regulatory constraints as 4G networks, and must therefore adopt a set of parameters enabling them to reproduce the n°2 4G frame format.
Conclusion
Since most deployments in Europe are in the FR1 spectrum, with numerology µ=1, we can expect latencies to be reduced in practice by a factor of 2 between 5G and 4G. It is interesting to note, however, that in 5G a significant further latency reduction is achievable, particularly in the millimeter spectrum, where numerologies 3 and 4 can be used. What's more, additional mechanisms such as mini-slots enable further latency reductions in certain use cases.
Finally, in the case of private 5G networks, it is possible to guarantee efficient interactions between terminals and the network, and therefore reduced application latency, through appropriate adjustment of configuration parameters.